Kleene algebra with tests is a beautiful, powerful framework for reasoning about programs. You can easily encode conventional While programs into KAT, and KAT enjoys decidable equality. Reasoning with KAT feels like you’re cheating Alan Turing himself: here we are, deciding nontrivial properties of programs!
The gist of KAT is that you write programs using a regular expression like notation: +
for parallel composition, ;
for sequential, and *
for iteration. So you might encode:
while x > 0:
y += 1
x -= 1
As (xGt0; incY; decX)*; ¬xGt0
, where xGt0
is a ‘test’ and incY
and decX
are ‘actions’. KAT’s equivalence decision procedure can prove that this program is equivalent to any finite unrolling of itself… neat!
NetKAT is the most impactful application of KAT: it’s an influential and successful academic project, and its ideas can already be found in numerous real, production systems. In light of NetKAT’s remarkable success… why don’t we apply KAT more often?
What’s hard about KAT?
On its own, KAT proves plenty of nice theorems, but none of them reason about particular program behaviors. In the code snippet above, xGt0
, incY
, and decX
are uninterpreted—there’s no relationship between, say xGt0
and decX
. That is, you might expect that ¬xGt0
;decX;¬xGt0
is equivalent to ¬xGt0;decX
, because decrementing a number less than or equal to 0 will yield a number that is also less than or equal to 0. The names of our tests and actions are suggestive, but KAT treats them absractly. If you want to reason about the semantics of your tests and actions, you need to build a custom, concrete KAT. NetKAT reasons about fields on packets, and doing so means building a particular, concrete KAT with particular actions. The original paper spends quite a bit of effort proving this new, custom KAT has a sound, complete, and decidable equivalence checking.
Worse still, KAT’s metatheory is very challenging. To create NetKAT, Nate Foster and company worked through closely related ideas for a few years before Nate joined Cornell and started working with Dexter Kozen, KAT’s progenitor. Only then did they realize that KAT would be a good fit, and they got to work on developing a concrete KAT—NetKAT. Unfortunately, “collaborate with Dexter” is an approach that doesn’t scale.
How to cook a KAT
In an upcoming PLDI 2022 paper, “Kleene Algebra Modulo Theories: A Framework for Concrete KATs”, Ryan Beckett, Eric Campbell, and I show how to generate a KAT over a given theory, i.e., a set of tests, actions, and their equational theory. We call the approach Kleene algebra modulo theories, or KMT. The paper covers quite a few examples:
- booleans and bit vectors
- monotonic natural numbers
- unbounded sets and maps
- NetKAT
What’s more, our approach allows for higher-order theories, like taking the product of two theories or using finite-time LTL to reason about another theory. (Our approach abstracts and generalizes Temporal NetKAT, which is just a concrete instance of our more general method.)
To build a KMT, you provide primitive tests and actions, along with weakest preconditions relating each pair of test and action. There’s an ordering requirement: a test must be no smaller than its preconditions. With these in hand, we’re able to automatically derive a KAT with good properties in a pay-as-you-go fashion:
- If your theory is sound, the KAT is sound.
- If your theory is complete, the KAT is complete.
- If your theory’s satisfiability checking is decidable, we can derive a decision procedure for equivalence.
I’m particularly excited that our framework is prototype-ready: our code is implemented as an OCaml library, where you define theories as functors. Please try it out—mess around and write your own theories, following our examples. We hope that KMT will significantly lower the bar for entry, making it easier for more people to play around with KAT’s powerful equivalence checking.
What’s the catch?
There’s more than one way to cook a KAT. KMT generates KATs with tracing semantics, i.e., the exact trace of actions matters. In KAT+B! or NetKAT, later updates override earlier ones, e.g., x:=false; x:=true ? x:=true
… but KMT will treat these terms differently, because they have different traces. KAT+B! deliberately avoids tracing; NetKAT only traces at predefined points, by means of their dup
primitive, which marks the current state as historically salient. There’s no deep reason for KMT to use tracing, and we believe KMT can be generalized to support dup
-like controls for tracing.
The ordering constraint on weakest preconditions is a strong one. Our natural numbers, sets, and maps must be monotonic: they may grow or shrink, but not both. They cannot be compared, e.g., two natural-valued variables x
and y
can be compared to constants but not each other.
KMT is also just a prototype. It’s fast for small programs, but it takes dedicated work to make a KAT’s decision procedure efficient enough on more serious examples.
Why are you talking about cooking KATs?
The greatest POPL paper of all time is Manna and Pnueli 1983, “How to cook a temporal proof system for your pet language”. Why? Just take a look a the first page:
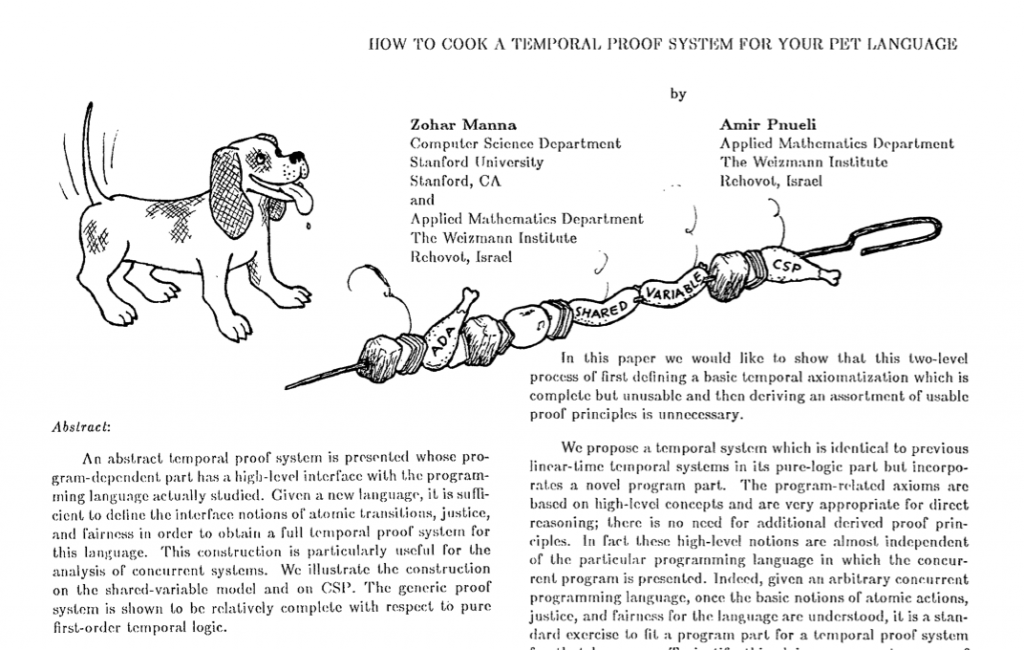
Update
KMT won a distinguished paper award at PLDI!